
HOW DOES COVID-19 SPREAD? THE CONTROVERSY AND THE EVIDENCE
Jessica Bartoszko and Mark Loeb | August 21, 2020
Last month, a letter titled “It is time to address airborne transmission of COVID-19”, signed by 241 scientists from 30 countries, was sent to the World Health Organization (WHO) and published in an infectious diseases journal (1). The letter appealed to the medical community and health regulatory bodies to recognize the potential for airborne spread of SARS-CoV-2. The letter emphasized that studies have demonstrated “beyond any reasonable doubt” that viruses are released during talking or coughing and remain small enough to travel distances beyond the 2 meters recommended by most physical distancing recommendations. The letter lamented the fact that, apart from aerosol generating procedures (AGPs) performed in healthcare settings, such airborne transmission is not recognized (1).
The publication of this letter led to a flurry of commentary in the media and not surprisingly polarized debate on social media. The implications are important. If airborne transmission is indeed widespread, this would mean most of the control strategies to reduce transmission of SARS-CoV-2 have been misguided. The publication of the letter has led to a vigorous response from infection disease physicians, challenging the premise of this letter (2).
An important question to ask therefore is “what is the scientific evidence to support airborne transmission for SARS-CoV-2?”
It is first important to define “airborne” and “droplet” transmission. Droplets refer to large particles (over 5 microns) which fall to the ground within 2 metres of the index case. Airborne particles, also referred to as droplet nuclei or aerosols, are smaller, and can therefore remain suspended in the air for longer and travel further distances (3). Certain medical procedures like bronchoscopy and intubation – the aforementioned AGPs – can generate these airborne particles and put healthcare workers at greater risk of infection; however, AGPs are unique to clinical settings (4,5). It is also important to note that although “airborne” and “droplet” are often presented as binary, the reality is there is a continuum of particle size that exists. People can be observed to generate respiratory particles of different sizes (6-10). The generation of airborne microdroplets has also been proposed as a transmission mechanism (11). Even a single cough can contain respiratory particles ranging from 0.1 to 100 microns in size (i.e. both aerosols and droplets) (10). Despite acceptance of variability in particle size, infectivity of these respiratory secretions also depends on viral load within, and the duration and route of exposure. Therefore, there is still debate about whether “droplet” versus “airborne” transmission predominates. This has manifested into conflicting recommendations on the use of personal protective equipment for healthcare workers against COVID-19 outside of AGPs. The WHO and Public Health Agency of Canada (PHAC) recommend medical (surgical) masks for non-AGP care of COVID-19 patients (12,13); whereas, the European Centre for Disease Prevention and Control (ECDC), and US Centers for Disease Control and Prevention (CDC) recommend N95 respirators (14,15). It is also important to note that contact transmission, that is contamination of hands or the environment, is also felt to be a possible risk (3). This is because other respiratory viruses, including coronaviruses, are transmitted this way; however, this mode of transmission has not yet been documented for SARS-CoV-2 (3). Precautions for respiratory infections in hospitals to date have generally included contact and droplet precautions.
Proving one mode of transmission over another with absolute certainty can be a daunting challenge. To a considerable extent, the weight placed on the various types of evidence is a function of the training and belief system of those making the determination. Aerosol scientists place the greatest weight on experiments such as instruments that generate aerosols (16,17), cough-chamber studies (18) or animal studies (19). It is important to note these experiments have important limitations as they often do not include infectious specimens, and use nebulizers or healthy people, to mimic SARS-CoV-2 respiratory secretions from coughs from sick individuals. The most obvious limitation is that none of these experiments demonstrate person-to-person airborne transmission (16-19). In contrast, clinical scientists and epidemiologists rely on epidemiological investigations. The majority of these, in both nonhealthcare and healthcare settings, are compatible with contact or droplet transmission, as determined by exposure patterns from source to recipient (20-25). One example is the investigation of the first community-acquired case of COVID-19 in the US. A total of 121 healthcare workers were exposed before this patient was recognized as being infected with SARS-CoV-2. Only 3 healthcare workers (2.5%) subsequently became infected. There was minimal transmission despite healthcare workers not donning appropriate personal protective equipment (only 1 of 3 infected wore a medical mask) and prolonged exposure to AGPs without N95 respirators (recommended protection for AGPs) (20). This investigation found that outside of nebulizer treatment (an AGP), unprotected, prolonged patient contact (e.g. performing a physical exam) was associated with infection in healthcare workers. These observations are inconsistent with “airborne” transmission. Further, a case-report of respiratory protective devices for COVID-19 in Singapore found there was no hospital-acquired transmission in 41 healthcare workers exposed to AGPs from a single patient – where only 6 healthcare workers wore N95 respirators (15%) (21). However, there are some exceptions, as investigations of outbreaks in a restaurant (26), choir practice (27) and cruise ship (28) suggested airborne transmission. Crowded indoor spaces with poor ventilation promote an increased burden of aerosols – aerosols would otherwise circulate in unimportant amounts. It is important to note, however, these outbreak investigations are not definitive as other routes of transmission, including contact and droplet transmission, or a superspreading event if compliance to hand hygiene and mask use were low, could not be ruled out (3,26-29).
While the aerosol scientists rely on controlled conditions in very artificial environments, clinical epidemiologists rely on clinical studies, including trials, that seek direct evidence of transmission but where controlling extraneous variables is more difficult. Although more direct and thus providing stronger inferences, it is often more difficult to discern between routes of transmission in epidemiological studies when data on physical proximity, the duration and intensity of the contact, and presence of AGPs is not captured. Such studies are bolstered by clinical experience whereas those of aerosol scientists are bolstered by physical properties of aerosols. Some simulation studies based on modelling suggest airborne transmission; however, these are difficult to interpret and are not based on data but on assumptions (28,30).
Infectious disease physicians rely on their experience and generally believe aerosol transmission as the predominant transmission mode to be highly unlikely, given the cases that they have seen collectively to date (3). In other words, if aerosol transmission was really predominant, they believe they would know by now. How would they? Physical distancing would certainly not work, and clusters of cases would arise where there was no obvious close contact with those infected. Small case studies to date are indeed suggestive of droplet spread, however these are uncontrolled studies and can be difficult to interpret (20, 21,25). Another argument is that the widespread use of surgical masks, which generally only protect against large droplets unlikeN95 respirators which block at least 95 percent of 0.3 micron particles, has not been associated with increase COVID-19 in healthcare workers (20,21). Further, low secondary rates of infection have been observed when people share meals (7%) (23), within households (13%) (23), and within hospitals (3%) (20). A higher incidence of infection would be expected if airborne transmission was a contributing factor. This is the case for measles, a viral infection for which transmission via aerosols is common.
From the perspective of clinical observation, it is impossible to “rule out” the possibility of airborne transmission as one would need access to every COVID-19, outbreak or cluster and having detailed epidemiologic data on each, which is not feasible. Clinical epidemiologists would agree, however, that under certain conditions aerosol transmission might occur (1). In the acute healthcare setting this could include the occurrence of an AGP, such as intubation or bronchoscopy or in a nursing home when ventilation is not functioning effectively.
In contrast, aerosol biologists cite the fact that people can generate respiratory particles of different sizes to support their point of view. However, such observations do not provide any direct support that airborne person-to-person transmission is common in either clinical or non-clinical settings. One piece of evidence they use is studies showing that COVID-19 viral RNA in the environment and demonstrate aerosolization of some respiratory particles. However, they were conducted in artificial environments and do not demonstrate transmission (16,17). These filter studies only demonstrate dispersal of nucleic acid, as most did not use culturable virus needed for infection. Even in the single study using cultured viable virus in aerosols, the viral load required to cause infection is unknown and this may or may not reflect what is occurring in healthcare facilities (17,31). As stated in a recent Canadian study “As it stands, demonstration of infectious virus from aerosol samples remains the most convincing evidence for transmission potential. Even if viral RNA were to become a reliable indicator of exposure, translating these results to manage and reduce risk requires significantly more research since little is known about transmission potential for given quantities of virus shed in the air” (32).
In summary, there is no strong evidence to support the idea that aerosol transmission is the most common mode of transmission for COVID-19. The recommendations of the aerosol biologists, however, are reasonable, in that they are “practical and often can be easily implemented; many are not costly”. Having adequate ventilation and sanitization are stressed and both the aerosol biologists and clinicians would agree with this. The recommendations include 1) proper ventilation, especially in indoor spaces like workplace environments, schools, hospitals and nursing homes; 2) supplementing general ventilation with airborne precautions (e.g. high efficiency air filtration) when possible; and 3) avoiding overcrowding, especially in indoor spaces (1). These recommendations, in addition to existing contact and droplet precautions, will thwart multiple mechanisms of SARS-CoV-2 transmission. Such bundled prevention strategies are important in controlling the spread of SARS-CoV-2 and preventing associated disease and death (1,3).
References
1. Morawska L, Milton DK. It is Time to Address Airborne Transmission of COVID-19. Clinical Infectious Diseases. 2020 Jul 6;ciaa939.
2. Chagla Z, Hota S, Khan S, Mertz D, and International Hospital and Community Epidemiology Group. Letter. Re: It is Time to Address Airborne Transmission of COVID-19. Submitted to CID.
3. World Health Organization. Transmission of SARS-CoV-2: implications for infection prevention precautions. 2020 July 9. Available online: https://www.who.int/publications/i/item/modes-of-transmission-of-virus-causing-covid-19-implications-for-ipc-precaution-recommendations
4. Durante-Mangoni E, Andini R, Bertolino L, Mele F, Bernardo M, Grimaldi M, et al. Low rate of severe acute respiratory syndrome coronavirus 2 spread among health-care personnel using ordinary personal protection equipment in a medium-incidence setting. Clin Microbiol Infect. 2020:S1198743X20302706.
5. Wong SCY, Kwong RTS, Wu TC, Chan JWM, Chu MY, Lee SY, et al. Risk of nosocomial transmission of coronavirus disease 2019: an experience in a general ward setting in Hong Kong. J Hosp Infect. 2020;105(2):119-27.
6. Morawska, L. et al. Size distribution and sites of origin of droplets expelled from the human respiratory tract during expiratory activities. Journal of Aerosol Science 2009; 40: 256-269.
7. Yan J, Grantham M, Pantelic J, et al. Infectious virus in exhaled breath of symptomatic seasonal influenza cases from a college community. Proceedings of the National Academy of Sciences of the United States of America USA, 2018; 115: 1081–6.
8. Xie, X., Li, Y., Chwang, A., Ho, P. & Seto, W. How far droplets can move in indoor environments--revisiting the Wells evaporation-falling curve. Indoor Air, 2007; 17: 211-225.
9. Lindsley, W.G. et al. Viable influenza A virus in airborne particles from human coughs. Journal of Occupational and Environmental Hygiene, 2015; 12: 107-113.
10. Jayaweera M, Perera H, Gunawardana B, Manatunge J. Transmission of COVID-19 virus by droplets and aerosols: A critical review on the unresolved dichotomy [published online ahead of print, 2020 Jun 13]. Environ Res. 2020;188:109819. doi:10.1016/j.envres.2020.109819
11. Youtube video from japan: 3. Coronavirus: New Facts about Infection Mechanisms - NHK Documentary. April 3, 2020. https://www.youtube.com/redirect?v=H2azcn7MqOU&event=video_description&q=https%3A%2F%2Fwww3.nhk.or.jp%2Fnhkworld%2Fen%2Fondemand%2Fvideo%2F%3Fcid%3Dwohk-yt-2004-corona03-hp&redir_token=awNHW-j8fp4vnYruT7ZXoFCZ8Z58MTU4Njc3NDQ3MkAxNTg2Njg4MDcy [Google Scholar]
12. World Health Organization (WHO). Rational use of personal protective equipment for coronavirus disease 2019 (COVID-19). April 6, 2020. https://www.who.int/publications/i/item/rational-use-of-personal-protective-equipment-for-coronavirus-disease-(covid-19)-and-considerations-during-severe-shortages. Accessed July 31, 2020.
13. Public Health Agency of Canada (PHAC). Coronavirus disease (COVID-19): For health professionals. July 27, 2020. https://www.canada.ca/en/public-health/services/diseases/2019-novel-coronavirus-infection/health-professionals.html. Accessed July 31, 2020.
14. Centers for Disease Control and Prevention (CDC). Interim Infection Prevention and Control Recommendations for Patients with Suspected or Confirmed Coronavirus Disease 2019 (COVID-19) in Healthcare Settings July 15, 2020. https://www.cdc.gov/coronavirus/2019-ncov/infection-control/control-recommendations.html. Accessed July 31, 2020.
15. European Centre for Disease Prevention and Control. Guidance for wearing and removing personal protective equipment in healthcare settings for the care of patients with suspected or confirmed COVID-19. February 2020. https://www.ecdc.europa.eu/sites/default/files/documents/COVID-19-guidance-wearing-and-removing-personal-protective-equipment-healthcare-settings-updated.pdf. Accessed July 31, 2020.
16. Van Doremalen N, Bushmaker T, Morris DH, Holbrook MG, Gamble A, Williamson BN, et al. Aerosol and surface stability of SARS-CoV-2 as compared with SARS-CoV-1. N Engl J Med. 2020;382:1564-7.
17. Fears AC, Klimstra WB, Duprex P, Weaver SC, Plante JA, Aguilar PV, et al. Persistence of Severe Acute Respiratory Syndrome Coronavirus 2 in Aerosol Suspensions. Emerg Infect Dis 2020;26(9).
18. Dudalski N, Mohamed A, Mubareka S, Bi R, Zhang C, Savory E. Experimental investigation of far-field human cough airflows from healthy and influenza-infected subjects [published online ahead of print, 2020 Apr 18]. Indoor Air. 2020;10.1111/ina.12680. doi:10.1111/ina.12680
19. Kim Y, Kim S, Kim S, Kim E, Park S, Yu K, et al. Infection and rapid transmission of sars-cov-2 in ferrets. Cell host & microbe. 2020.
20. Heinzerling A, Stuckey MJ, Scheuer T, Xu K, Perkins KM, Resseger H, et al. Transmission of COVID-19 to Health Care Personnel During Exposures to a Hospitalized Patient — Solano County, California, February 2020. MMWR Morb Mortal Wkly Rep. 2020 Apr 17;69(15):472–6
21. Ng K, Poon BH, Kiat Puar TH, Shan Quah JL, Loh WJ, Wong YJ, et al. COVID-19 and the Risk to Health Care Workers: A Case Report. Annals of Internal Medicine. 2020 Jun 2;172(11):766–7.
22. Maogui Hu, Hui Lin, Jinfeng Wang, Chengdong Xu, Andrew J Tatem, Bin Meng, Xin Zhang, Yifeng Liu, Pengda Wang, Guizhen Wu, Haiyong Xie, Shengjie Lai, The risk of COVID-19 transmission in train passengers: an epidemiological and modelling study, Clinical Infectious Diseases, , ciaa1057, https://doi.org/10.1093/cid/ciaa1057
23. Chen Y,Wang AH, Yi B, et al. Epidemiological characteristics of infection in COVID-19 close contacts in Ningbo. Article in Chinese
24. Chen J, He H, Cheng W, Liu Y, Sun Z, Chai C, et al. Potential transmission of SARS-CoV-2 on a flight from Singapore to Hanghzou, China: An epidemiological investigation. Travel Medicine and Infectious Disease. 2020 Jul;36:101816.
25. Park SY, Kim Y-M, Yi S, Lee S, Na B-J, Kim CB, et al. Coronavirus Disease Outbreak in Call Center, South Korea. Emerg Infect Dis [Internet]. 2020 Aug [cited 2020 Jul 13];26(8). Available from: http://wwwnc.cdc.gov/eid/article/26/8/20-1274_article.htm non healthcare setting
26. Li, Y. et al. Evidence for probable aerosol transmission of SARS-CoV-2 in a poorly ventilated restaurant. medRxiv, 2020; https://www.medrxiv.org/content/10.1101/2020.04.16.20067728v1 (accessed 05/06/2020).
27. Hamner L, Dubbel P, Capron I, et al. High SARS-CoV-2 Attack Rate Following Exposure at a Choir Practice — Skagit County, Washington, March 2020. MMWR Morb Mortal Wkly Rep 2020;69:606–610. DOI: http://dx.doi.org/10.15585/mmwr.mm6919e6external icon 28.
28. Azimi P, Keshavarz Z, Cedeno Laurent JG, Stephens BR, Allen JG. Mechanistic Transmission Modeling of COVID-19 on the Diamond Princess Cruise Ship Demonstrates the Importance of Aerosol Transmission. medRxiv 2020.07.13.20153049; doi: https://doi.org/10.1101/2020.07.13.20153049 29.
29. Adam D, Wu P, Wong J, Lau E, Tsang T, Cauchemez S, et al. Clustering and superspreading potential of severe acute respiratory syndrome coronavirus 2 (SARS-CoV-2) infections in Hong Kong (pre-print). Research Square. 2020. doi: 10.21203/rs.3.rs-29548/v1 30.
30. Feng, Y., Marchal, T., Sperry, T., & Yi, H. (2020). Influence of wind and relative humidity on the social distancing effectiveness to prevent COVID-19 airborne transmission: A numerical study. Journal of aerosol science, 147, 105585. Advance online publication. https://doi.org/10.1016/j.jaerosci.2020.105585
31. Bullard J, Dust K, Funk D, Strong JE, Alexander D, Garnett L, et al. Predicting infectious SARS-CoV-2 from diagnostic samples. Clin Infect Dis. 2020:ciaa638.
32. Yip, L., Finn, M., Granados, A., Prost, K., McGeer, A., Gubbay, J. B., Scott, J., & Mubareka, S. (2019). Influenza virus RNA recovered from droplets and droplet nuclei emitted by adults in an acute care setting. Journal of occupational and environmental hygiene, 16(5), 341–348. https://doi.org/10.1080/15459624.2019.1591626
This article initially appeared in the Globe and Mail on August 12, 2020.
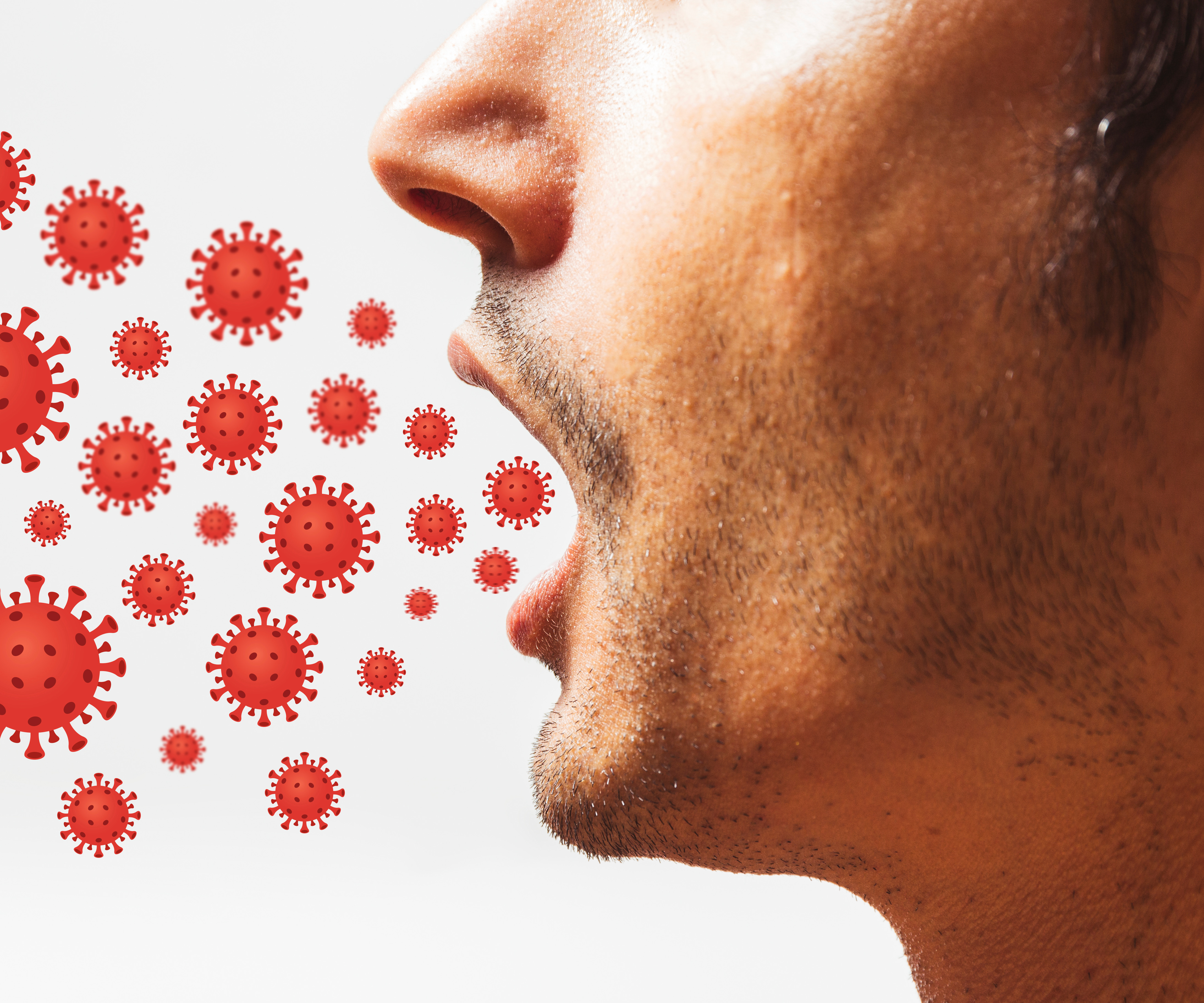