Under what conditions did prebiotic organic compounds form and how did they polymerise? When did life first evolve, what was it, and how did it metabolise? How has biological innovation through time impacted the composition of the Earth’s surface? These are just some of today’s many unresolved questions, and for many, we have only scratched the surface. What we do know, however, is that life must have begun under conditions considerably different from today. Imagine an environment with surface temperatures approaching 100oC; a steamy, smoggy atmosphere with over 1000 times the present level of carbon dioxide, and high levels of gases such as nitrogen, sulphur dioxide, elemental sulphur, and carbon monoxide. There would have been virtually no O2, and as a result, little ozone to attenuate the intense incoming UV radiation. An expansive ocean, far different from the one we know today, would have existed with abundant ferrous iron, silica and ammonium. No continents, at least in the modern sense, and perhaps not even exposed land. On top of all this, the surface was subjected to a heavy bombardment by extra-terrestrial material. This was the Hadean Earth, from 4.4-4.0 billion years ago (Ga), and it would have been extremely inhospitable to life as we know it, but it is nonetheless, the most likely time when the beginnings of life took place.
Supporting evidence for this journey back in time comes from an extremely limited suite of ancient geological samples. Analyses based on the chemical and isotopic composition of their minerals and organic remains, the few putative Archean microfossils, and some potential organosedimentary structures, have unfortunately led to interpretations fraught with controversy. Each of these indicators for Earth’s earliest life can simultaneously be explained by invoking abiogenic mechanisms or contamination by younger material, while a single, definitive piece of evidence for early life remains elusive. Coupled to the chemical evidence is the extrapolation of molecular information from modern microorganisms. But there again, incorporating those findings into phylogenetic trees has proven equally contentious and led to highly ambiguous results. Yet, a convergence of “biosignatures” suggests that an active biosphere dominated by an assortment of bacteria existed by at least 3.2 Ga, if not earlier. By 2.5 Ga, there is indisputable evidence for increasingly complex life. This interval in Earth’s history is often referred to as the Great Oxidation Event (GOE), and it represents the first time that free oxygen (as O2 gas) accumulated into the atmosphere. A remarkably coherent ensemble of evidence points to this oxygenation event, including the disappearance of detrital pyrite, uranitite, and siderite from fluvial and deltaic deposits, an increase in the retention of ferric iron in paleosols, and an enrichment of Cr and U in iron formations. Perhaps most telling though is the disappearance of mass-independent sulphur isotope anomalies (S-MIF), indicative of atmospheric SO2 processing and the absence of an appreciable ozone layer, from the sedimentary record. From a biological perspective, this event indicates that oxygen-producing microorganisms, the precursors to modern cyanobacteria, must have existed at this time.
Research amongst my group at the University of Alberta is focused on using ancient sedimentary rocks, such as banded iron formations (BIF), as proxies for early life. The premise is that BIF allow us to track a wide variety of bioessential trace elements that act as structural components and reactive centres in metalloenzymes and are required by life. These requirements differ between organisms and have evolved over geological time, likely guided in some part by environmental conditions. Until recently, most of our understanding regarding trace element concentrations in Precambrian oceans came from extrapolation, geochemical modelling, and/or genomic studies. In the past decade, however, the increasing availability of trace element and isotopic data from sedimentary rocks of all ages have yielded new, and potentially more direct, insights into secular changes in seawater composition – and ultimately the evolution of the marine biosphere. Compiled records of many trace elements (Ni, Mo, P, Zn, Co, Cr, U, Se, and I) are providing a first glimpse at how trace element availability in Earth’s ancient oceans may have affected biological evolution. Several of these trace elements display redox-sensitive behaviour (e.g., Mo, I), while others are redox-sensitive but not bioessential (e.g., Cr, U). Temporal trends in sedimentary archives are providing useful constraints on changes in atmosphere-ocean redox conditions that are inherently linked to biological evolution, specifically the activity of cyanobacteria. Three examples are provided here. First, it has been proposed that low phosphorous (P) concentrations in Archean (4.0-2.5 Ga) BIF reflected limited marine P availability, which would have reduced levels of photosynthesis, cyanobacterial growth, and carbon burial, thereby inhibiting long-term oxygen production on the early Earth. A subsequent increase in the P content of BIF in the Neoproterozoic (1.0-0.5 Ga), following Snowball Earth deglaciations, may have led to enhanced cyanobacterial photosynthesis, and produced enough oxygen to facilitate the evolution of animal life. Second, it has been shown that the nickel (Ni) content in BIF has changed dramatically over time, driven by a decrease in oceanic Ni availability around 2.7 billion years ago that likely had profound consequences for methane-producing bacteria. These bacteria, methanogens, have a unique Ni requirement for their methane-producing enzymes, and crucially, methanogens have been implicated in controlling oxygen levels on the ancient Earth as the methane they produce would have reacted with oxygen, keeping atmospheric oxygen levels low. It is possible that a Ni famine led to a cascade of events, beginning with reduced methane production, the expansion of previously marginalized cyanobacteria into shallow-water settings, and culminating with increased oxygenic photosynthesis that tipped the atmospheric balance in favour of oxygen, resulting in the GOE at 2.5 Ga. Third, a recent compilation of Cr enrichment in BIF shows a profound enrichment coincident with the GOE. Given the insolubility of Cr minerals, its mobilisation and incorporation into BIF indicates enhanced chemical weathering by oxygen, most likely associated with the onset of terrestrial aerobic pyrite oxidation. It is in this manner that the trace element contents of BIF provide us with a powerful window into Earth’s early oceans.
Kurt Konhauser is a Professor in the Department of Earth and Atmospheric Science at the University of Alberta and a world leader in Geobiology. Recently, he has used his biogeochemical knowledge to infer the composition of Earth’s surface environment, particularly the timing of its oxygenation.
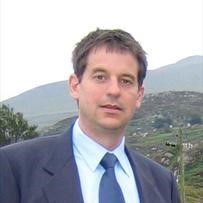